Stem Cells from Fat Tissue Protect Motor Neurons in ALS Mice
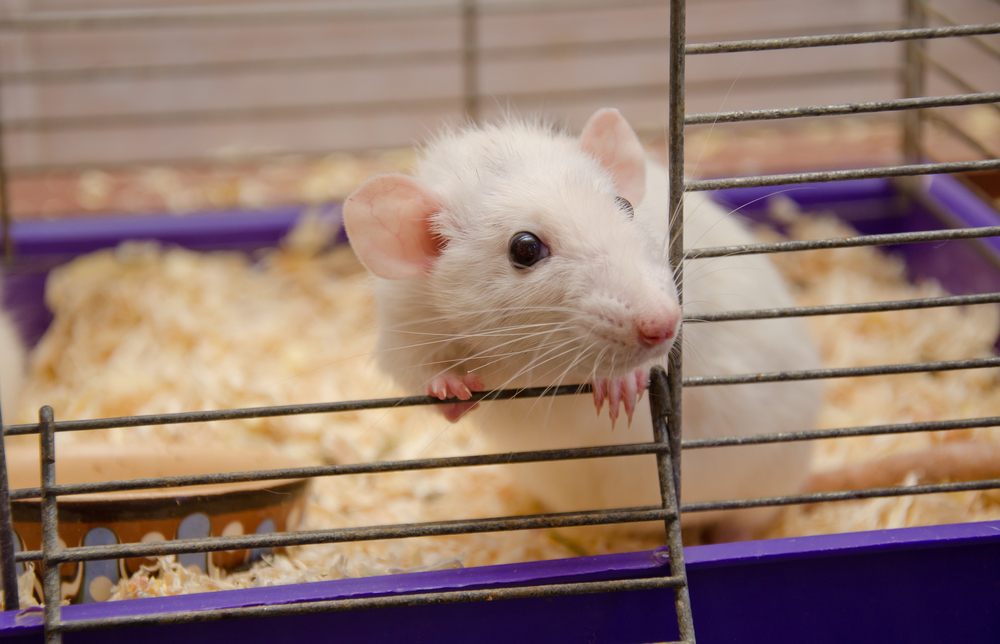
Stem cells derived from fat tissue significantly improved motor function and delayed disease onset by protecting motor neurons in a mouse model of amyotrophic lateral sclerosis (ALS), a study shows.
The study, “Adipose derived stem cells protect motor neurons and reduce glial activation in both in vitro and in vivo models of ALS,” was published in the journal Molecular Therapy, Methods & Clinical Development.
Stem cell therapy represents a potential therapeutic approach to treat ALS. Mesenchymal stem cells (MSCs) are a type of adult stem cell found mainly in bone marrow, giving rise to various cell types, including bone cells, muscle cells, and fat cells (adipocytes).
MSCs show low immune rejection, can modulate the immune system, and secrete cell growth factors to help support and protect nearby cells. Studies show that transplanting MSCs into an ALS mouse model improves motor function, reduces nerve cell inflammation, slows disease progression, and extends lifespan.
However, the primary source of MSCs comes from aspirating bone marrow, which is invasive, expensive, and provides a limited number of cells. Adipose tissue may represent an alternative source of MSCs — called adipose-derived stem cells (ADSCs) — that can be harvested by less invasive procedures such as liposuction, are more abundant, and show more growth potential compared to bone marrow-derived MSCs.
In this study, a team of investigators at the University of Sheffield in the U.K. tested the impact of mouse-derived ADSCs in cell- and animal-based models of ALS.
Mouse ADSCs were isolated from fat tissue of healthy mice and injected into the fluid that surrounds the brain and spinal cord (intrathecal injection) of SOD1-G93A mice, an animal model of the disease that carries a mutation in the SOD1 gene.
Mutations in the SOD1 gene account for up to 20% percent of familial ALS cases, and SOD1-G93A mice show a significant decline in motor function as early as 40 days of age, before visible signs of the disease emerge. Mice aged 30 to 32 days were assigned randomly to receive ADSC treatment or a saline solution as a control.
Mice treated with ADSC injection showed a significant increase in body weight compared with the control group, from 60 days of age to the end of the study at 90 days. Treated mice also performed better on the rotarod test, a measure of motor function, compared with control mice.
Motor function decline was delayed significantly in ADSC-transplanted mice by approximately two weeks and delayed visible signs of disease by five days. Additionally, ADSC-treated mice showed significant improvements in walking ability. An examination of spinal cord tissue obtained from 90-day-old mice showed that ADSC-injected mice had significantly more motor neurons — the nerve cells damaged in ALS that control voluntary muscle movements.
In ALS, damage to motor neurons is amplified by the activation of non-neuronal cells such as astrocytes, which support neurons, and microglial cells, a type of immune cell. Spinal cord tissue also found that astrocyte and microglial activation were reduced significantly in ADSC-treated mice than control mice.
“These results are in agreement with a beneficial clinical effect on motor neuron survival associated with a reduction in the spinal cord inflammatory milieu mediated by astrocyte and microglial activation,” the team wrote.
Next, astrocytes were isolated from SOD1-G93A mice (or controls) and co-cultured with motor neurons from healthy mice along with ADSCs to test whether the results in mice were directly linked to ADSC-mediated protection against altered astrocytes.
After seven days, there was a significant reduction in motor neurons in the SOD1-G93A astrocyte cultures compared with controls, “confirming that SOD1-G93A astrocytes are toxic and/or less able to support [motor neuron] survival,” the team added. In contrast, the presence of ADSCs in the SOD1-G93A culture resulted in a significant increase of about 23% in motor neuron viability compared with untreated cultures, “abolishing the toxicity.”
A proposed mechanism by which ADSCs protect nerve cells is through the secretion of growth factors such as VEGF and IGF-1. VEGF secretion in ADSCs was increased following culture with control astrocytes as well as SOD1-G93A astrocytes.
Furthermore, culture with SOD1-G93A astrocytes, but not with control astrocytes, induced ADSCs to secrete significantly higher levels of IGF-1. ADSC treatment also increased the secretion of VEGF in control astrocytes, but not in ALS-mice astrocytes, while enhancing the secretion of IGF-1 in both control and SOD1G93A astrocytes. In particular, there was a fivefold increase in IGF-1 secretion in SOD1-G93A astrocytes.
ALS astrocytes also exert neural toxicity by secreting pro-inflammatory proteins (cytokines). Here, SOD1-G93A astrocytes secreted significantly higher pro-inflammatory cytokine interleukin 6 (IL-6) levels than control astrocytes. ADSCs reduced the secretion in this and other cytokines.
Finally, the team grew motor neurons together with astrocytes derived from ALS patients (with familial or sporadic ALS). As expected, motor neuron survival in ALS-derived astrocyte cultures was reduced compared with controls. The presence of mouse ADSCs protected motor neurons from both sporadic and familial ALS astrocytes.
“Our results indicate that a single intrathecal dose of ADSCs injected into SOD1G93A mice at the presymptomatic stage is able to significantly improve motor performance and delay disease onset through motor neuron protection and attenuation of glial activation,” the investigators wrote. “These data provide evidence that ADSCs possess similar therapeutic properties to those of [bone marrow]-MSCs.”